Time integrated mass flux samplers (TIMs): An estimate of sediment flux
In the hydrological, geomorphological and aquatic sciences, information regarding the quality and quantity of fine fluvial suspended sediment transferred through the river network is of vital importance for a number of reasons: i) in determining the life expectancy of dams (Graf, Wohl et al. 2010); ii) assessing the magnitude of sediment removal and associated pathogens from agricultural lands (Walling, Russell et al. 2002); iii) gauging the effects of river restoration, land management practices and land-use change on sediment delivery (Downs and Kondolf 2002); assessing the health of our rivers with reference to legislature (Collins and Anthony 2008) and iv) evaluating habitats and biodiversity.
In order to determine the suspended sediment load of a river, two observable parameters are essential. These are the instantaneous values of suspended sediment concentration and river discharge. The latter is relatively accessible in most drainage basins of the UK given that the EA currently maintain over 1000 gauging stations. These stations typically collect high frequency flow measurements recorded at 15-minute intervals. However, suspended sediment concentration data is somewhat more difficult to acquire given the lack of well equipped sediment monitoring schemes in the UK. Where monitoring schemes do exist, their ability to accurately attain good quality suspended sediment data is largely dependent on two key issues.
The first issue is the choice of method which is used to acquire the suspended sediment flux measurement. The chosen method can appreciably alter the representativeness of the sample concentration and the frequency at which samples can be taken. There are a range of widely used methods for generating suspended sediment flux data, which range from simple, direct and manually operated techniques to more complex, indirect and automated methods.
Given that up to 90% of annual fine suspended sediment loads can be transported in under 10% of time, the second determinant on the quality of suspended sediment flux data is the sampling scheme that is adopted. The experimental design must be capable of recording the temporal discontinuity in fine sediment transfer, maximising confidence in the suspended sediment flux estimates, whilst being cost-effective. This is a difficult balance to attain given that confidence intervals of estimates may be viewed as a function of the number of samples (Dixon and Chiswell 1996).
In order to maximise the efficiency of monitoring campaigns, numerous different sampling strategies have been developed including time and flow proportional sampling, probability sampling and stratified sampling. However, each of these approaches is greatly limited by their temporal sampling resolution over the course of a year, with load estimates typically requiring the use of interpolation procedures which may not yield sufficiently accurate fine suspended sediment load estimates. Although, these direct measurements of suspended sediment flux do physically capture the sediment being transferred, the mass collected from a typical sample volume is rarely enough for physical or geochemical analysis (> 10g) except during the most severe events.
An alternative approach which is widely adopted as a means of the flux of fine sediment in river channels is the use of turbidity as a proxy for suspended sediment concentrations. This is a particularly attractive alternative given that it is relatively easy to measure at high frequencies. Although turbidity is not a quantitative measure of suspended sediment concentrations, it is a proxy which has been shown to be strongly correlated to in-stream suspended sediment concentrations (Gippel 1995; Lenhart, Brooks et al. 2010). The utilisation of this technique is often limited to outlet monitoring due to the level of investment required to setup and maintain monitoring sites. Furthermore, this approach also fails to provide information about the physical properties of the fine sediment in transport.
Given the issues described with regard to the aforementioned approaches a potentially attractive alternative is to deploy time-integrated sampling apparatus which continuously collect a mass of fine sediment that is representative of the flux, physical and geochemical characteristics of the ambient transported material. According to Nelson & Benedict (1951), such sampling devices should be isokinetic, pointed into the flow, protrude upstream of the area of disturbance, be movable, suitable for transport, streamlined, not drift downstream, rugged, simple to construct and inexpensive. A sampler which meets these criteria has the potential to provide a means of monitoring the suspended sediment flux of a river at locations distributed throughout a catchment.
An example of a time integrated sampling device is the Time Integrated Mass- flux sampler (TIMs), designed by Phillips et al. (2000). The TIMs is subject to the full range of flow conditions and sediment fluxes over the sampling period, providing a continuous record fine sediment flux, which may be representative of all events (Walling et al., 2008). This apparatus is often used for capturing a sufficient mass of fine fluvial suspended sediment for sediment fingerprinting (e.g. Fox and Papanicolaou 2007; Collins, Walling et al. 2010; Fukuyama, Onda et al. 2010; Martínez-Carreras, Krein et al. 2010) and has been used as a means of estimating the relative suspended sediment yield of a river (Bolland, Bracken et al. 2010).
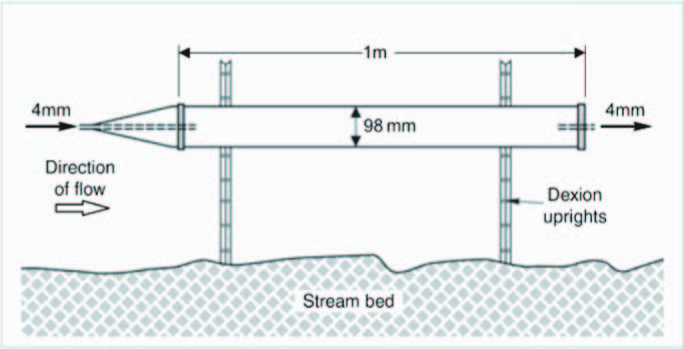
Given the relatively minimal financial outlay required to set up and maintain a distributed network of these samplers, it may be possible to densely instrument a catchment in this way. Such an experimental design would: i) provide a relative assessment of the suspended sediment flux across a catchment; ii) provide catchment managers with data required to highlight areas in a catchment responsible for the delivery of fine suspended sediment; iii) monitor the effectiveness of measures targeted at reducing fine sediment flux; iv) produce a mass of sediment which can subsequently be used for source ascription work e.g. sediment fingerprinting; v) produce data which can be used to test distributed transport models e.g. SCIMAP
References:
Bolland, J. D., L. J. Bracken, et al. (2010). “A protocol for stocking hatchery reared freshwater pearl mussel Margaritifera margaritifera.” Aquatic Conservation: Marine and Freshwater Ecosystems 20(6): 695-704.
Collins, A. L. and S. G. Anthony (2008). “Assessing the likelihood of catchments across England and Wales meeting ‘good ecological status’ due to sediment contributions from agricultural sources.” Environmental Science & Policy 11(2): 163-170.
Collins, A. L., D. E. Walling, et al. (2010). “Assessing damaged road verges as a suspended sediment source in the Hampshire Avon catchment, southern United Kingdom.” Hydrological Processes 24(9): 1106-1122.
Dixon, W. and B. Chiswell (1996). “Review of aquatic monitoring program design.” Water Research 30(9): 1935-1948.
Downs, P. W. and G. M. Kondolf (2002). “Post-Project Appraisals in Adaptive Management of River Channel Restoration.” Environmental Management 29(4): 477-496.
Fox, J. F. and A. N. Papanicolaou (2007). “The use of carbon and nitrogen isotopes to study watershed erosion processes.” Journal of the American Water Resources Association 43(4): 1047-1064.
Fukuyama, T., Y. Onda, et al. (2010). “Quantifying the impact of forest management practice on the runoff of the surface-derived suspended sediment using fallout radionuclides.” Hydrological Processes 24(5): 596-607.
Gippel, C. J. (1995). “Potential of turbidity monitoring for measuring the transport of suspended solids in streams.” Hydrological Processes 9: 83 – 97.
Graf, W. L., E. Wohl, et al. (2010). “Sedimentation and sustainability of western American reservoirs.” Water Resour. Res. 46(12): W12535.
Lenhart, C., K. Brooks, et al. (2010). “Spatial and temporal variation in suspended sediment, organic matter, and turbidity in a Minnesota prairie river: implications for TMDLs.” Environmental Monitoring and Assessment 165(1): 435-447.
Martínez-Carreras, N., A. Krein, et al. (2010). “A rapid spectral-reflectance-based fingerprinting approach for documenting suspended sediment sources during storm runoff events.” Journal of Soils and Sediments 10(3): 400-413.
Nelson, M. E. and P. C. Benedict (1951). Measurement and analysis of suspended sediment stream loads in streams, ASCE. Volume 116: 891 – 918.
Phillips, J. M., M. A. Russell, et al. (2000). “Time-integrated sampling of fluvial suspended sediment: a simple methodology for small catchments.” Hydrological Processes 14: 2589 – 2602.
Walling, D. E., A. L. Collins, et al. (2008). “Tracing suspended sediment and particulate phosphorus sources in catchments.” Journal of Hydrology 350(3-4): 274-289.
Walling, D. E., M. A. Russell, et al. (2002). “Establishing sediment budgets for two small lowland agricultural catchments in the UK.” CATENA 47(4): 323-353.